The use of Biodegradable Polymers is growing in the world to reduce the impact of plastic on the environment. There are various raw materials used to make the polymers biodegradable. However, each one of them has their own pros and cons. The ultimate goal of the packaging is to store the content packed in it till its shelf life and degrade naturally when disposed off in the environment. This makes it viable and sustainable for use without impacting the life forms in the eco-system. LCA is a useful tool to evaluate whether a product or process is eco-friendly.

Benefits of LCA
- LCA helps to select and compare the products or processes with least environmental impact. This information is useful when combined with other factors like cost and performance to select the right product or process
- LCA allows to study an entire product system. Thus, chances of sub-optimization are significantly reduced
- LCA determines the effects on one or more environmentally sensitive locations.
- LCA helps to discover significant product enhancements and novel approaches to process optimization. In some cases, it results in developing a new product or service to cater to the same need.
Phases of LCA
LCA assessment comprises of the following 4 phases depicted in Fig 2:
- Goal and Scope Definition,
- Inventory Analysis,
- Impact Assessment,
- Interpretation
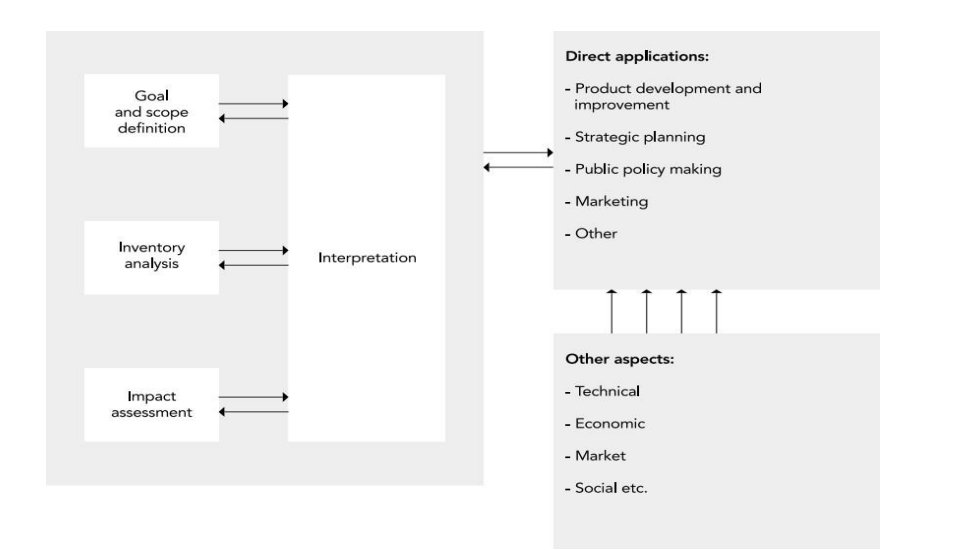
Fig 2: Phases of LCA (1)
- Goal and Scope: The goal of the study is to evaluate the environmental impacts associated with a conventional LLDPE film, and a Biodegradable LLDPE film. In the LCA study, GaBi software is used to model the life cycle of Biodegradable LLDPE film, to make the inventory and to estimate the related environmental burdens following ISO 1440 and 1444 standards.
- Inventory Analysis: It is the identification, collection and calculation of inputs and outputs of environmental flows across the system boundaries. The raw materials, in process intermediates, the finished materials, electricity and water consumption are thoroughly studied, recorded and demonstrated for additive, masterbatch as well as film manufactured using the Biodegradable Additive. The findings are subjected to Impact Assessment using the Gabi Software.
- Impact Assessment: The impact assessment phase of an LCA assigns the results of the inventory analysis to different impact categories(1) . The impact categories considered in the study are shown in Fig 3, and some of the selected categories are included in the study, namely:
- Global Warming Potential (GWP or Carbon Footprint)
- Abiotic resource depletion
- Acidification
- Eutrophication
- Ozone layer depletion
- Photochemical oxidation
- Human toxicity
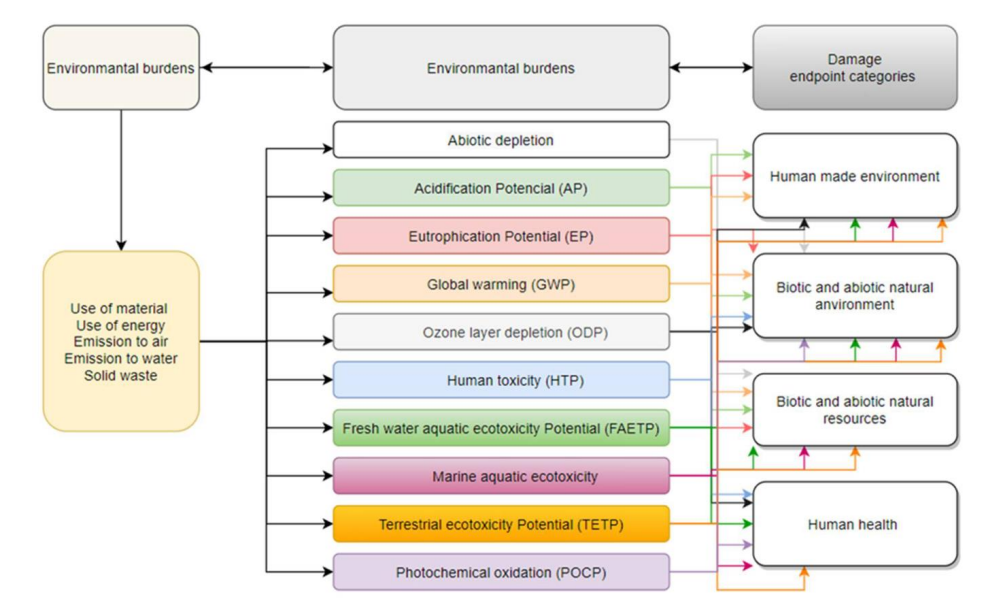
Fig 3: Impact Categories in LCA (1)
- Global warming (GWP): Global warming potential (GWP) is a measure of how much of a given mass of a greenhouse gas (for example, CO2, methane, nitrous oxide) is estimated to potentially contribute to global warming. It is measured in terms of CO2
- Abiotic depletion: It refers to the depletion of non-living (abiotic) resources such as fossil fuels, minerals, metals, clay and peat. It is measured in kilograms of Antimony (Sb) equivalents.
- Ozone Layer depletion (ODP): Changes to atmospheric ozone will modify the amount of harmful UV radiation penetrating the earth’s surface with potential adverse effects on human health and ecosystems. It is measured in terms of CFC equivalents.
- Photochemical Oxidation (POCP): It includes the complex reactions between NOx and VOCs in presence of sunlight resulting in formation of ozone in the troposphere. It is measured in terms of Ethylene equivalent.
- Eutrophication (EP): This is caused by the addition of nutrients largely line nitrogen and phosphorus to a soil or water system which leads to an increase in biomass, damaging other lifeforms. It is measured in terms of Phosphates (PO4-3) equivalents.
- Acidification (AP): It is the deposition of acids causing a decrease in the pH, a decrease in the mineral content of soil and increased concentrations of potentially toxic elements in the soil solution. The major acidifying pollutants are SO2, NOx, HCL and NH3. It is measured in terms of SO2
- Toxicity: It is the degree to which something is able to produce illness or damage to an exposed organism. There are 3 different types of toxicity; human toxicity (HTP), terrestrial ecotoxicity (TEP), and fresh water aquatic ecotoxicity (FAEP). It is measured in terms of dichlorobenzene equivalents.
LCA Findings of Alternative Polymer Packaging
Several studies have been conducted to ascertain viability and sustainability of alternative packaging solutions to plastic. Few findings about PLA, Bio-PET, PBS, Bio-PE, SBS and CA extracted from the literature reviews are as summarized below.
PLA (Polylactic acid)
PLA is made from lactic acid. Lactic Acid is produced through the fermentation of dextrose typically sourced from corn, however any starch-rich feedstock could be used. Manufacturing of PLA is an energy-intensive process. The LCA studies of PLA indicate that it emits a significant quantity of CO2 into the environment. More than 50% (2.8 kg CO2 per kg PLA) of the CO2 is released during the life cycle of PLA is due to its conversion. It requires significant amount of land, water and energy for production making it unviable and non-sustainable (2)(3).
Bio-PET (Polyethylene Terephthalate)
Bio-PET is considered one of the most significant bioplastics. It is partially produced from renewable sources. However, it’s production is not yet economically viable. There are few studies available in the literature for bio-PET, but they are performed on lab scale batches. These have limitations as compared to the batches of large scale production. These LCA studies indicate that bio-PET plastic is highly resistant to biodegradation due to its high aromatic content. This promotes its accumulation in the environment (2)(3).
Polybutylene succinate (PBS)
Polybutylene succinate (PBS) is a thermoplastic polyester. It can be produced from food waste. PBS has properties similar to polyethylene terephthalate (PET) and polypropylene (PP). The LCA analysis of PBS is based on GHG emissions, land acidification, and fine particulate matter production. GHG emissions from producing PBS from food waste are approximately 5.88 kg CO2-eq per kg of PBS (2)(3).
Bio-PE (Polyethylene)
Bio-PE is currently produced utilizing first-generation ethanol derived from food crops such as sugarcane. Ethanol is then catalytically dehydrated to yield ethylene. This ethylene is subsequently either polymerized to yield PE or oxidized to yield ethylene oxide. It is then hydrolyzed to yield bio-based mono-ethylene glycol (bio-MEG) which is the bio-based component of bio-PET. One kilogram of bio-PE costs around 30% more than 1 kg of fossil-based PE. Some LCA studies on Bio-PE report that the production of bio-PE leads to 140% less GHG emissions as compared to the production of petrochemical PE. The savings on the use of non-renewable energy are approximately 65%. If biomass is used as a steam source for the manufacturing process, it can further minimize GHG emissions (2)(3).
Starch-based Biopolymer (SBP)
Starch is a polymer derived from different feedstocks such as potato, corn, or wheat. It is a polysaccharide composed of two major components: amylopectin and amylose. The non-edible lignocellulosic biomass of the feedstock raw materials is used for SBP production. SBPs are a promising class of bioplastics. The LCA studies of SBPs showed significantly lower impact in terms of both NREU (Non-renewable energy use) and GWP (Global warming potential) than that of petrochemical polymers. However, it was recognized that these SBPs could not compete with recycled petrochemical polymers (2)(3).
Cellulose acetate (CA)
CA is a semi-synthetic polymer. It is a commonly used, chemically modified natural polymer. It is mostly derived from cellulose through acetylation of some hydroxyl groups and is an eco-friendly material. Cellulose is primarily derived from wood through the pulping process. It may be transformed into a variety of compounds such as rayon, cellophane, cellulose esters and cellulose ethers. The primary mechanisms responsible for the degradation of cellulose-based polymers are chemical and biological hydrolysis. The LCA studies of CA show that it can be biodegraded or hydrolyzed after consumption into cellulose and acetic acid in the natural environment. These compounds return to the environment with no adverse effects (2)(3).
Above findings from the literature indicate that there is a significant need to evaluate Bioplastic Sustainability Pillars. At present, it is difficult to determine definitively whether Bioplastics are more practical and environmentally friendly than Petroleum-based plastics because there is a lack of data. Additionally, it is difficult to determine what benefits and/or drawbacks Bioplastics may cause as a feedstock for the long-term advancement of a sophisticated circular bioeconomy. This subject requires thorough research going forward to enable multiple sustainable solutions. Till the, LCA (Life Cycle Assessment) will certainly be a useful tool to identify more sustainable methods of production, use and disposal of bio-based products (2)(3).
LCA findings of NICHEM’s Biodegradable Additive
The LCA analysis of the Biodegradable Additive developed by NICHEM was performed by NITIE (National Institute of Industrial Engineering, now recognized as IIM-Indian Institute of Management) using GaBi software to study the environmental impact of various impact categories described previously in this article. It was observed that significant impact was coming from the usage of electricity during the drying process during manufacturing of the additive. Similarly, during the production of the film, the use of LLDPE polymer also significantly contributed in different impact categories. The present study highlighted the environmental impact of Biodegradable LLDPE film in comparison with only LLDPE film without the active additive. It was clearly observed that, the impact of the conventional LLDPE films (without additive) was significantly higher than the Biodegradable LLDPE film in both landfill as well as incineration disposal conditions. The GWP was higher in conventional LLDPE film. In case of Biodegradable LLDPE film there was no need to be incinerated because of its degradation ability. Thus, the Biodegradable film showed no harmful impact on the environment (1).
Further, possibility of use of alternative energy resources (natural gas, biogas, and solar) was studied to reduce the environmental impact due to coal energy. The impact of replacing coal energy was found significant after the use of other resources. The least environmental impact was observed from the solar panel, followed by biogas and natural gas. In comparison to coal gas, significant impact could be reduced by almost 50% in solar panel. Hence, the replacement of coal based energy by solar panel would reduce the overall environmental burden proving it to be a sustainable solution (1).
Till the policymakers make a disposal pathway for Bioplastics, use of Biodegradable Additives like BioX in polymers is certainly a viable alternative which can be considered for effectively managing plastic waste, especially the flexible packaging.
Why replace when you can degrade effectively?
To know more, please visit our website www.nichem.solutions
References:
- Life Cycle Assessment (LCA) of BIOX Report (2022), National Institute of Industrial Engineering (NITIE), now known as IIM (Indian Institute of Management); pg 1-5.
- Sameh Samar Ali et al (2023), Bioplastic production in terms of life cycle assessment: A state-of-the-art review; Environmental Science and Ecotechnology; 15 (2023)100254; 1-21.
- Troy A Hottle et al (2013), Sustainability Assessments of Bio-based polymers; Polymer Degradation and Stability; 98 (2013) 1898-1907